The Symphony Within: Unveiling the Secrets of Nerve Communication
A Journey Through the Brain's Hidden Network
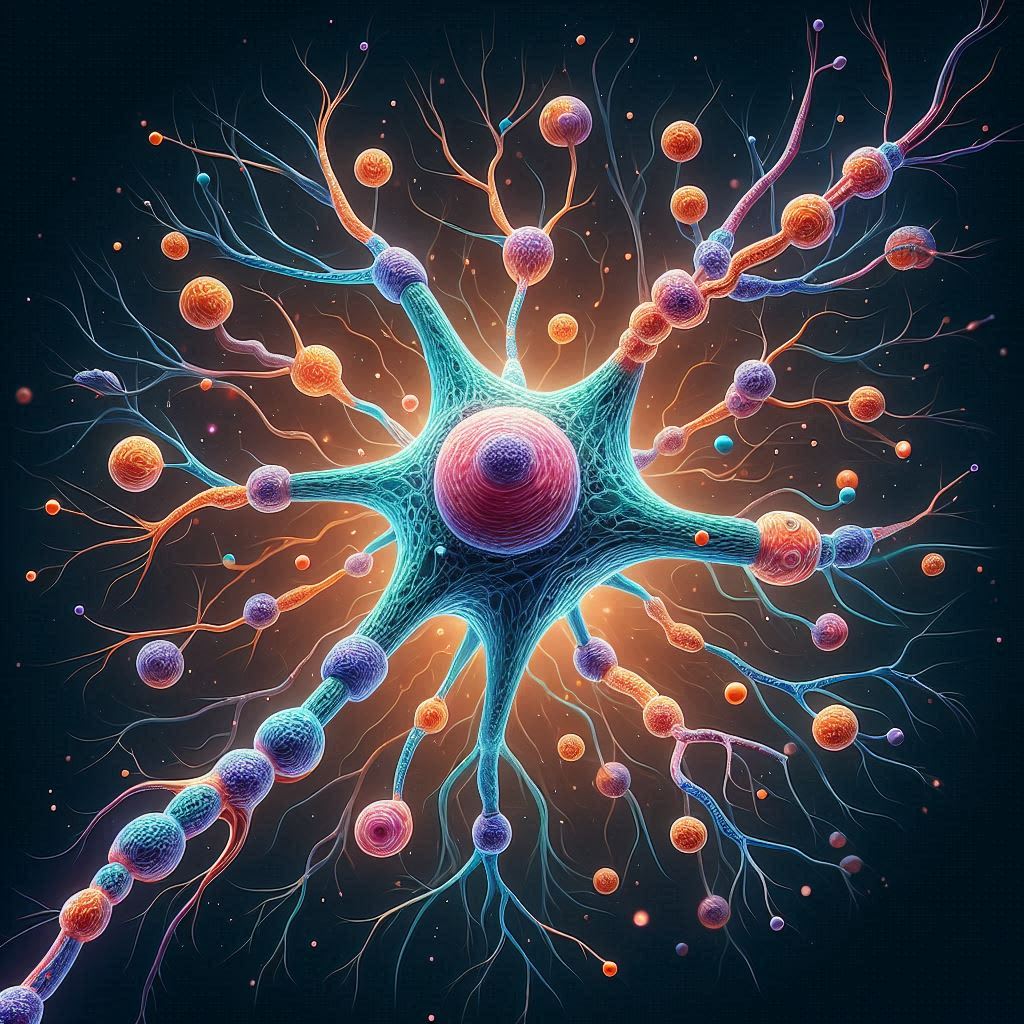
The Hidden Symphony Within: Unveiling the Marvels of Nerve Cell Communication
Imagine a bustling metropolis, a symphony of activity orchestrated by a complex network. This isn't a city of steel and glass, but the intricate world within your body – your nervous system. Billions of nerve cells, called neurons, act as the city's communication network, sending and receiving electrical signals that govern everything from muscle movement to the subtlest emotional nuance. But how do these microscopic marvels achieve such remarkable feats? Let's delve into the fascinating ultrastructure of nerves, exploring their building blocks and the intricate dance that allows us to think, feel, and move.
The Nerve Cell: A Master of Communication
Neurons come in various shapes and sizes, but all share a common architecture. The cell body serves as the command center, housing the nucleus and protein-making machinery. From here, a web of branching dendrites extends outward, acting like tiny antennas that receive signals from other neurons. These incoming messages travel down a single, long fiber called the axon, the information highway of the nervous system.
The magic of efficient communication lies in a special fatty sheath called myelin. This insulating layer, produced by glial cells (the city's support staff), surrounds the axon like electrical tape. Myelin isn't continuous, but rather segmented with gaps called nodes of Ranvier. This unique structure allows for a fascinating phenomenon known as saltatory conduction, where electrical impulses jump from node to node, significantly accelerating signal transmission.
At the axon's tip lies the axon terminal, the communication hub. Here, the electrical signal is converted into a chemical message in the form of neurotransmitters. These specialized molecules bridge the gap between neurons, diffusing across a tiny space called the synapse and triggering a response in the receiving neuron.
Classification: Form Follows Function
Neurons can be classified based on their structure and function. Unipolar neurons, with a single process emerging from the cell body, are rare and found in the ear. Pseudounipolar neurons, like sensory neurons, have a single axon that branches into two, allowing them to receive information from the environment (like the prick of a pin) and relay it to the central nervous system. Bipolar neurons, present in the retina, have one axon and one dendrite, facilitating a two-way flow of information between photoreceptor cells and other neurons in the visual pathway. The most common type, multipolar neurons, like motor neurons, have a single axon and numerous dendrites, enabling them to integrate signals from many sources (touch, balance, etc.) before sending a coordinated motor response to contract a muscle.
Functionally, neurons fall into three broad categories:
- Sensory neurons: These sentinels of the body gather information from the environment (touch, temperature, pain, etc.) and carry it towards the central nervous system. They typically have small axons and a pseudounipolar structure.
- Motor neurons: These serve as the commanders, carrying instructions from the brain and spinal cord to our muscles, dictating movement. They boast larger axons and a multipolar structure.
- Intermediate neurons: These information brokers reside within the central nervous system, integrating signals from various sensory neurons and directing them to motor neurons or other brain regions. For example, an intermediate neuron might receive information about a hot stovetop from a sensory neuron in your fingertip and then send a signal to a motor neuron in your arm, triggering a withdrawal reflex.
Beyond the Basics: A Glimpse into the Complexities of Nerve Communication
The world of nerve communication is far more intricate than the simple relay race we've explored so far. Here are some additional layers of complexity that orchestrate the symphony within:
- Neurotransmitters: These chemical messengers come in a diverse array, each with unique effects. Some, like dopamine, are associated with pleasure and reward, motivating us to repeat pleasurable activities (think delicious food or a social connection). Others, like acetylcholine, are crucial for muscle movement. Understanding the intricate interplay between different neurotransmitters holds the key to treating conditions like Parkinson's disease, where dopamine levels decline, and Alzheimer's disease, which involves disruptions in acetylcholine signaling.
- Neuromodulators: These chemicals don't directly trigger action potentials but rather fine-tune the overall communication process. They can amplify or dampen ongoing signals, allowing for a more nuanced and adaptable response. For instance, norepinephrine, a neuromodulator, can enhance alertness and focus when needed.
- Synaptic Plasticity: The connections between neurons aren't static; they can be strengthened or weakened through experience. This is the foundation of learning and memory formation. By repeatedly practicing a skill (like playing a musical instrument), the synapses involved in coordinating those movements become more efficient, allowing for faster and stronger signal transmission, leading to improved performance.
These intricate mechanisms are constantly under investigation, with scientists uncovering new facets of nerve communication all the time.
The Symphony's Conductors: The Role of Glial Cells
While neurons take center stage in communication, they rely heavily on a supporting cast – glial cells. Outnumbering neurons by ten to one, these unsung heroes perform a variety of essential functions:
- Myelination: As mentioned earlier, glial cells (oligodendrocytes in the CNS and Schwann cells in the PNS) are responsible for producing and maintaining the myelin sheath, ensuring efficient signal conduction. Damage to myelin, as seen in multiple sclerosis, disrupts communication and can lead to a range of neurological symptoms like muscle weakness, tremors, and vision problems.
- Nutrient Supply: Glial cells help regulate the delivery of nutrients and oxygen to neurons, providing the fuel they need to function optimally. They form a network of tiny tubes around blood vessels and neurons, facilitating the exchange of essential molecules.
- Waste Removal: They also play a critical role in clearing waste products generated by neuronal activity, maintaining a clean environment for optimal communication. This includes the removal of neurotransmitters after they've delivered their message, ensuring clear and efficient signaling between neurons.
- Immune Defense: Glial cells act as the immune system's first line of defense within the nervous system, protecting neurons from pathogens and damage. They can identify and eliminate invading bacteria or viruses, and also produce scar tissue to wall off damaged areas, preventing further spread of injury.
Understanding the diverse functions of glial cells is crucial not only for a comprehensive understanding of nerve communication but also for developing therapies for neurological disorders that involve glial dysfunction. For instance, research is underway to explore ways to stimulate the production of new myelin-forming cells as a potential treatment for multiple sclerosis.
The Power of the Microbiome: An Unexpected Influence
The trillions of microbes that reside within our gut, collectively known as the gut microbiome, might seem far removed from the nervous system. However, emerging research suggests a fascinating connection. The gut microbiome can influence the production of certain neurotransmitters, particularly those involved in mood regulation, and potentially modulate the activity of neurons in the brain-gut axis. This newfound understanding opens doors for exploring the potential role of gut health in neurological disorders like anxiety and depression. Studies are investigating whether interventions like probiotic supplementation or fecal microbiota transplants (FMT) could offer new avenues for treating these conditions.
Why This Matters?
By delving deeper into the complexities of nerve communication, we not only gain a deeper appreciation for the marvels of the human body but also unlock new avenues for therapeutic interventions. Understanding how neurotransmitters interact, the role of synaptic plasticity, and the influence of the gut microbiome can pave the way for the development of personalized treatments for a wide range of neurological conditions.
The Cutting Edge: Unveiling the Future of Nerve Science
The exploration of nerve science is a continuous journey. As research progresses, we can expect even more exciting discoveries about how our nervous system orchestrates our thoughts, feelings, and actions. Here are some frontiers at the forefront of this field:
- Brain-Computer Interfaces (BCIs): These devices hold immense promise for individuals with paralysis or sensory loss. By translating brain signals into computer commands, BCIs could enable people to control prosthetic limbs, communicate through text, or even interact with virtual environments. Recent advancements in BCI technology have shown remarkable progress, with individuals with paralysis achieving some degree of independent movement or communication through these devices.
- Optogenetics: This innovative technique utilizes light to control the activity of specific neurons. By genetically engineering neurons to be sensitive to light, scientists can activate or inhibit them with precise timing. Optogenetics offers a powerful tool for dissecting the complex circuits within the brain and has the potential to be used in future therapies for neurological disorders.
- Gene Editing for Neurological Conditions: The revolutionary CRISPR gene editing technology holds immense promise for treating a variety of genetic neurological disorders. By correcting faulty genes in nerve cells, scientists hope to one day be able to cure conditions like Huntington's disease or cystic fibrosis. However, significant ethical considerations and technical hurdles remain before widespread clinical applications become a reality.
Overall, the field of nerve science is brimming with exciting possibilities. As we continue to unravel the intricate workings of the nervous system, we inch closer to a future where neurological disorders become treatable, and the potential of the human brain is further unlocked.
About the Creator
suren arju
Hi there! I'm Suren, your startup guide. Entrepreneur, writer, dreamer - I share insights, tips & stories to fuel your startup journey. Ready to explore, learn & win together? Join me & let's redefine how we launch, learn & leap!
Enjoyed the story? Support the Creator.
Subscribe for free to receive all their stories in your feed. You could also pledge your support or give them a one-off tip, letting them know you appreciate their work.
Comments (1)
Suren, that symphony was incredible.