Biomass to Biochar: Full Life Cycle Carbon Footprint Analysis
Assessing Environmental Impact from Feedstock to Application
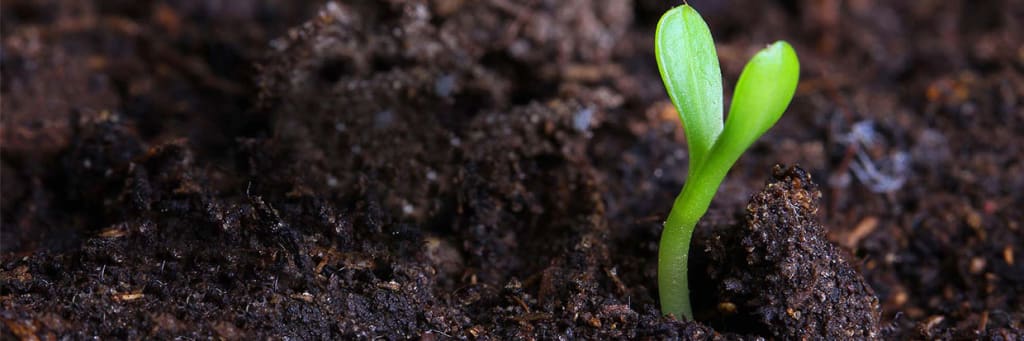
The conversion of biomass to biochar represents a promising strategy for carbon sequestration and soil enhancement. This process not only mitigates greenhouse gas emissions but also offers agricultural benefits. A comprehensive life cycle carbon footprint analysis provides insight into the environmental impacts of biochar production and use. By examining each stage, from biomass collection to biochar application, we can better understand the potential of this technology in achieving sustainability goals.
Biomass Collection and Transport
The first stage in the life cycle of biochar production involves the collection and transport of biomass. Common sources of biomass include agricultural residues, forestry waste, and dedicated energy crops. Each type of biomass has unique characteristics that influence its carbon footprint.
Agricultural Residues
Agricultural residues, such as corn stover, rice husks, and wheat straw, are abundant and often underutilized. Utilizing these residues for biochar production can reduce waste and improve soil health. However, the carbon footprint associated with their collection and transport must be considered. Fuel consumption by machinery and vehicles, as well as emissions from these activities, contribute to the overall carbon footprint.
Forestry Waste
Forestry waste, including logging residues and sawmill by-products, is another valuable source of biomass. Using forestry waste for biochar production can mitigate the environmental impact of forestry operations. The transport of this biomass, often from remote locations, can result in significant carbon emissions. Optimizing transport logistics and using energy-efficient vehicles can help reduce this footprint.
Energy Crops
Dedicated energy crops, such as switchgrass and miscanthus, are grown specifically for bioenergy production. These crops can be cultivated on marginal lands, reducing competition with food crops. The carbon footprint of energy crops includes emissions from land preparation, planting, cultivation, and harvesting. Sustainable agricultural practices and efficient use of inputs can minimize these emissions.
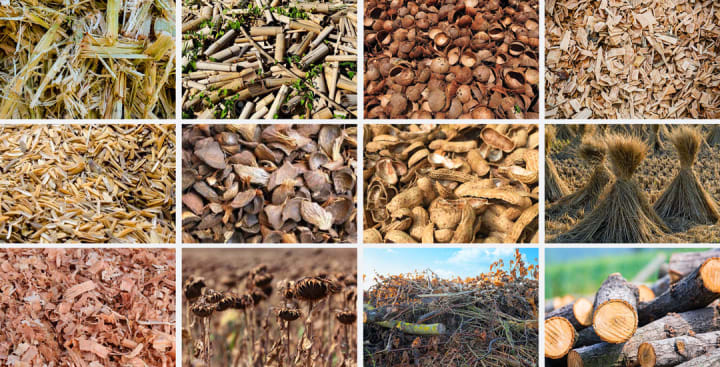
Pyrolysis Process
The core of biochar production is the pyrolysis process, where biomass is thermally decomposed in the absence of oxygen. This process produces biochar, syngas, and bio-oil. The efficiency and carbon footprint of the pyrolysis process depend on several factors, including temperature, residence time, and reactor design.
Temperature and Residence Time
The pyrolysis process typically operates at temperatures between 300°C and 700°C. Higher temperatures generally increase the production of syngas and bio-oil while reducing biochar yield. The residence time, or the duration biomass remains in the reactor, also affects the composition and quality of the products. Shorter residence times favor biochar production, whereas longer times increase the yield of gaseous and liquid products.
Reactor Design
The design of the pyrolysis reactor influences the efficiency and carbon footprint of the process. Common reactor types include fixed-bed, fluidized-bed, and rotary kiln reactors. Each type has specific advantages and limitations in terms of heat transfer, residence time, and scalability. The energy source used to heat the reactor, whether it be fossil fuels or renewable energy, significantly impacts the carbon footprint.
Energy Recovery
The syngas produced during pyrolysis can be used to generate heat and power, making the process more energy-efficient and reducing its carbon footprint. By utilizing the syngas on-site, the need for external energy inputs is minimized. This integration of energy recovery systems enhances the overall sustainability of biochar production.
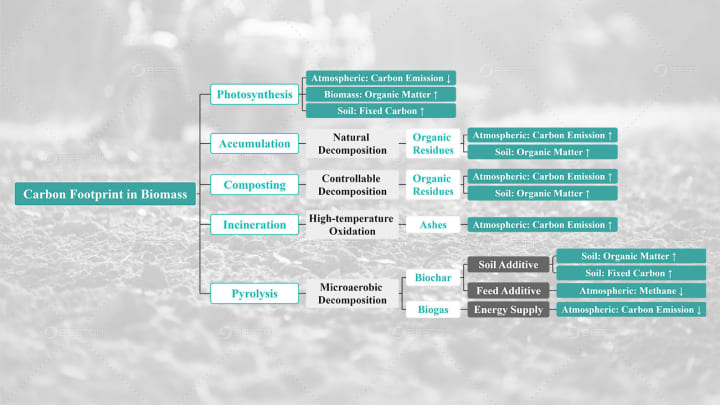
Biochar Application and Benefits
Biochar application to soil provides numerous environmental and agricultural benefits, contributing to its overall positive carbon footprint.
Carbon Sequestration
One of the most significant benefits of biochar is its ability to sequester carbon. When biomass is converted to biochar, a substantial portion of the carbon is stabilized in a solid form, preventing it from being released as carbon dioxide (CO2) into the atmosphere. This carbon can remain in the soil for hundreds to thousands of years, acting as a long-term carbon sink.
Biochar enhances soil health by improving its physical, chemical, and biological properties. It increases soil porosity, water retention, and nutrient availability, promoting healthier plant growth. Biochar also enhances microbial activity, contributing to soil fertility and resilience. These benefits can lead to higher crop yields and reduced need for chemical fertilizers, further decreasing the agricultural carbon footprint.
Reduced Emissions
The application of biochar to soil can reduce greenhouse gas emissions from agricultural activities. Biochar can decrease nitrous oxide (N2O) emissions, a potent greenhouse gas, by enhancing soil aeration and reducing the conditions that promote N2O formation. Additionally, biochar can reduce methane (CH4) emissions from rice paddies and other anaerobic environments.
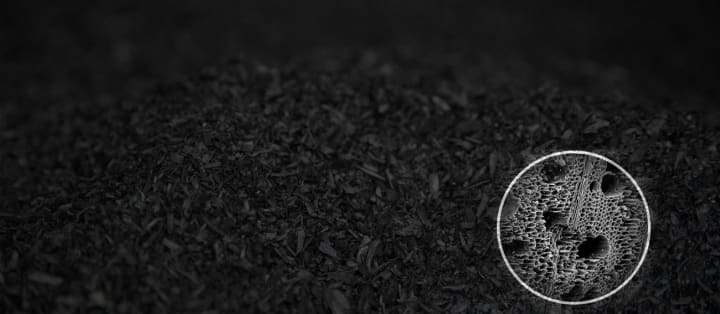
Life Cycle Assessment (LCA) of Biochar Production
A comprehensive LCA of biochar production involves evaluating the environmental impacts of each stage, from biomass collection to biochar application. This assessment considers the carbon emissions, energy use, and potential benefits associated with the entire process.
Carbon Emissions
Carbon emissions from biochar production primarily arise from biomass collection, transport, and the pyrolysis process. The type of biomass, distance transported, and energy source for pyrolysis all influence the total emissions. Integrating renewable energy sources and optimizing logistics can significantly reduce the carbon footprint.
Energy Use
The energy consumption of biochar production includes the energy required for biomass preparation, reactor operation, and product handling. The use of syngas for process heat can offset some of this energy demand. Additionally, implementing energy-efficient technologies and practices can further enhance the sustainability of biochar production.
Net Carbon Balance
The net carbon balance of biochar production is determined by comparing the carbon sequestered in the biochar to the carbon emissions associated with its production. If the carbon sequestered exceeds the emissions, the process is considered carbon-negative. This means that biochar production not only mitigates carbon emissions but also contributes to overall carbon reduction.
Sensitivity Analysis
Sensitivity analysis helps identify the key factors influencing the carbon footprint of biochar production. Variables such as biomass type, transport distance, pyrolysis temperature, and energy source can significantly impact the results. Understanding these sensitivities allows for the optimization of biochar production to maximize its environmental benefits.
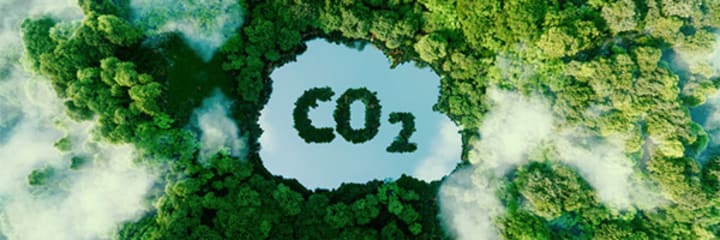
Economic and Policy Considerations
The economic viability of biochar production and the implementation of supportive policies are crucial for its widespread adoption.
Economic Viability
The economic feasibility of biochar production depends on several factors, including feedstock availability, production costs, and market demand for biochar and its co-products. Utilizing low-cost or waste biomass can enhance profitability. Additionally, the agricultural benefits of biochar, such as improved crop yields and reduced input costs, can provide economic incentives for farmers.
Policy Support
Supportive policies and regulations are essential to promote biochar production and application. Government incentives, such as subsidies, grants, and carbon credits, can reduce financial barriers and encourage investment in biochar technology. Policies that recognize biochar as a carbon sequestration method and promote its use in agriculture can drive its adoption.
Market Development
Developing markets for biochar and its co-products is crucial for the economic sustainability of biochar production. Biochar can be used in various applications, including soil amendment, water filtration, and construction materials. Expanding these markets can create new revenue streams and enhance the overall value proposition of biochar.
Conclusion
The full life cycle carbon footprint analysis of biomass to biochar production highlights its potential as a sustainable solution for carbon sequestration and soil enhancement. From biomass collection to biochar application, each stage of the process offers opportunities to enhance environmental and economic benefits. By leveraging technological innovations, supportive policies, and market development, biochar production can play a crucial role in achieving a sustainable and resilient future.
About the Creator
Enjoyed the story? Support the Creator.
Subscribe for free to receive all their stories in your feed. You could also pledge your support or give them a one-off tip, letting them know you appreciate their work.
Comments
There are no comments for this story
Be the first to respond and start the conversation.